Research
Population dynamics
The study of how interactions among species lead to changes in their populations -- where species may refer to animals and plants in a forest, or
microbes in a culture, or mutations and wild-type genes in gene
clusters, etc. -- is fundamental for understanding a number of fields like ecology, evolution and genetics (and its applications extend naturally to others like finance and demography). The last few decades have made it abundantly clear that these ostensibly biological systems can be studied very fruitfully by invoking physical methods and insights, rooted in the ideas of non-equilibrium dynamics.
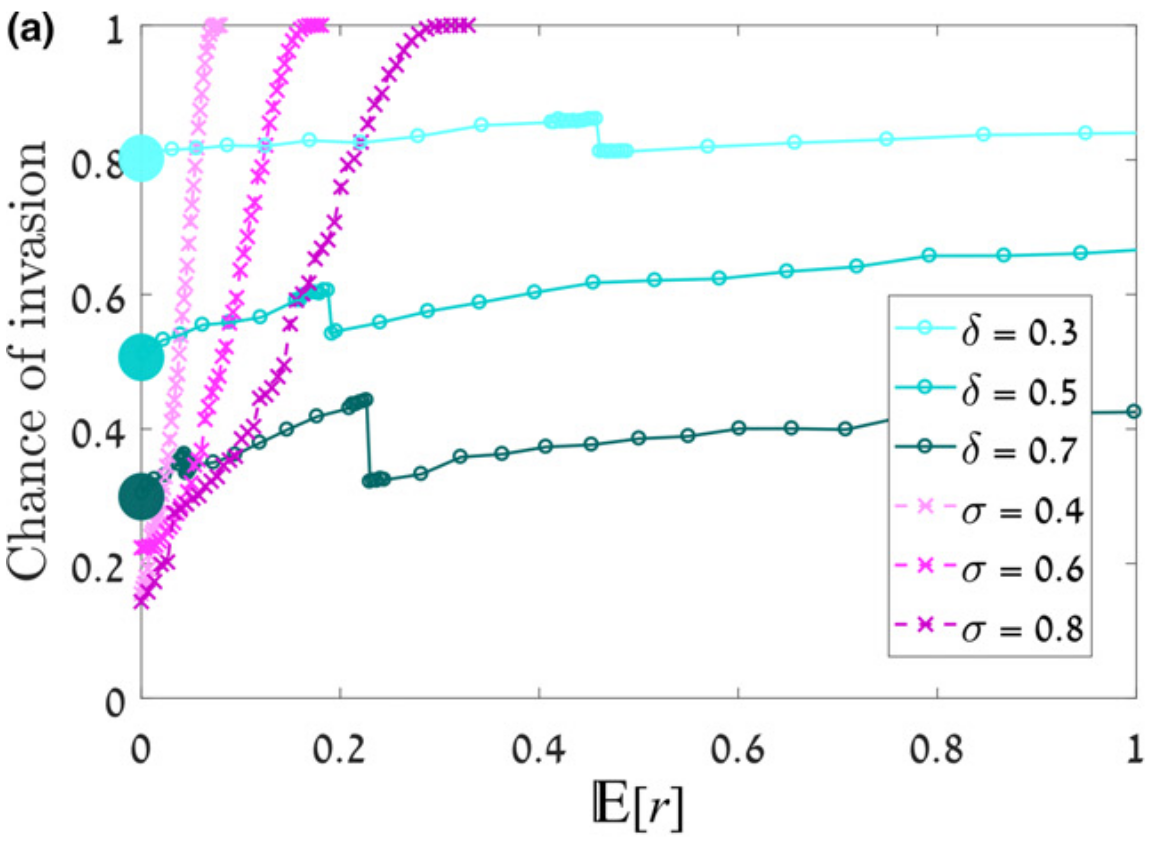
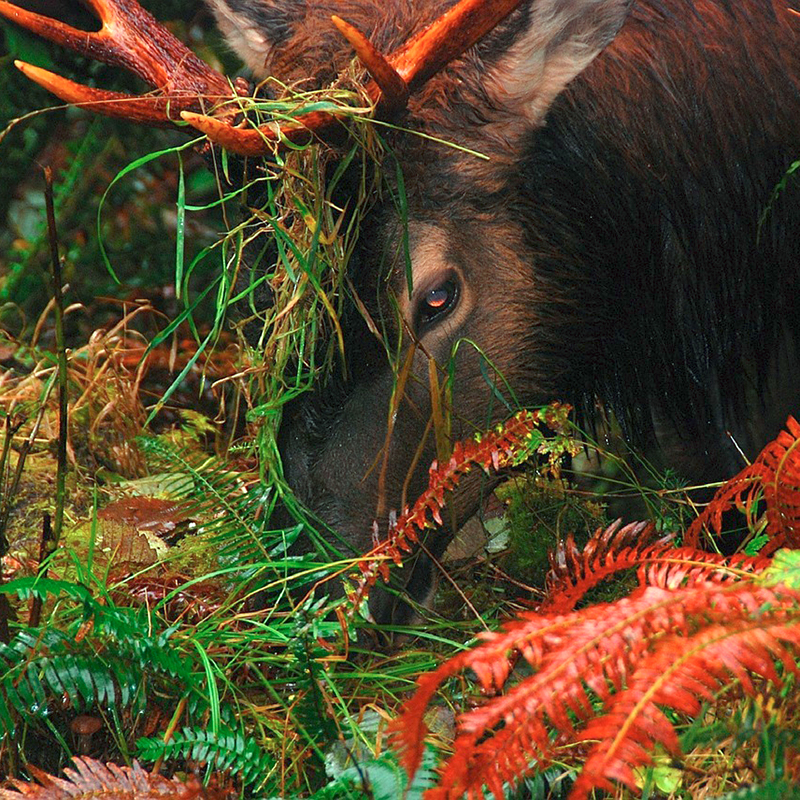
Much
of my current work focuses on widening our current theoretical
understanding of some fundamental concepts in population dynamics such
as the chance of invasion of a mutant species, the mean time to
extinction of a resident species, etc. We recently showed that contrary
to widespread belief, the mean growth rate of a species when it is rare
does not act as a reliable quantitative measure of the
persistence of a species in systems with fluctuating environments. We
are currently working, using analytical and numerical means, to define
new metrics which do do the job and have wide applicability.
See further:
Mean growth rate when rare is not a reliable metric for persistence of species, Jayant, T. Fung, R.A. Chisholm and N.M. Shnerb, Ecology Letters 23, 274 (2020). DOI:10.1111/ele.13430
Taming the diffusion approximation through a controlling-factor WKB method, Jayant and N.M. Shnerb, Physical Review E 102, 062410 (2020). DOI:10.1103/PhysRevE.102.062410
Microswimming
Microswimmers are microscopic bodies that can execute autonomous motion through fluids. Understanding and controlling their motion is of great significance for both biology -- with bacteria and other microbes forming ubiquitous examples of such swimmers -- and technology, with vital applications such as minuscule medical probes and lab-on-a-chip devices. Unfortunately, the heavily overdamped
nature of their surroundings in practically all real fluids
renders the motion of microswimmers complicated and necessitates fiendishly clever strategies of
motion that Nature has evolved over millenia but human ingenuity is still grappling to comprehend.
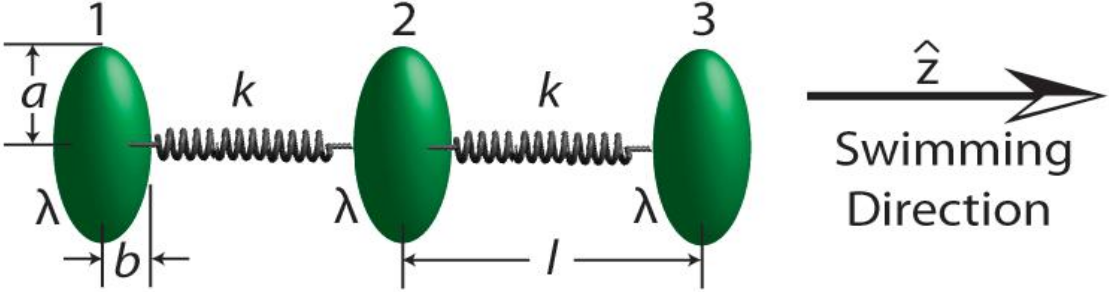
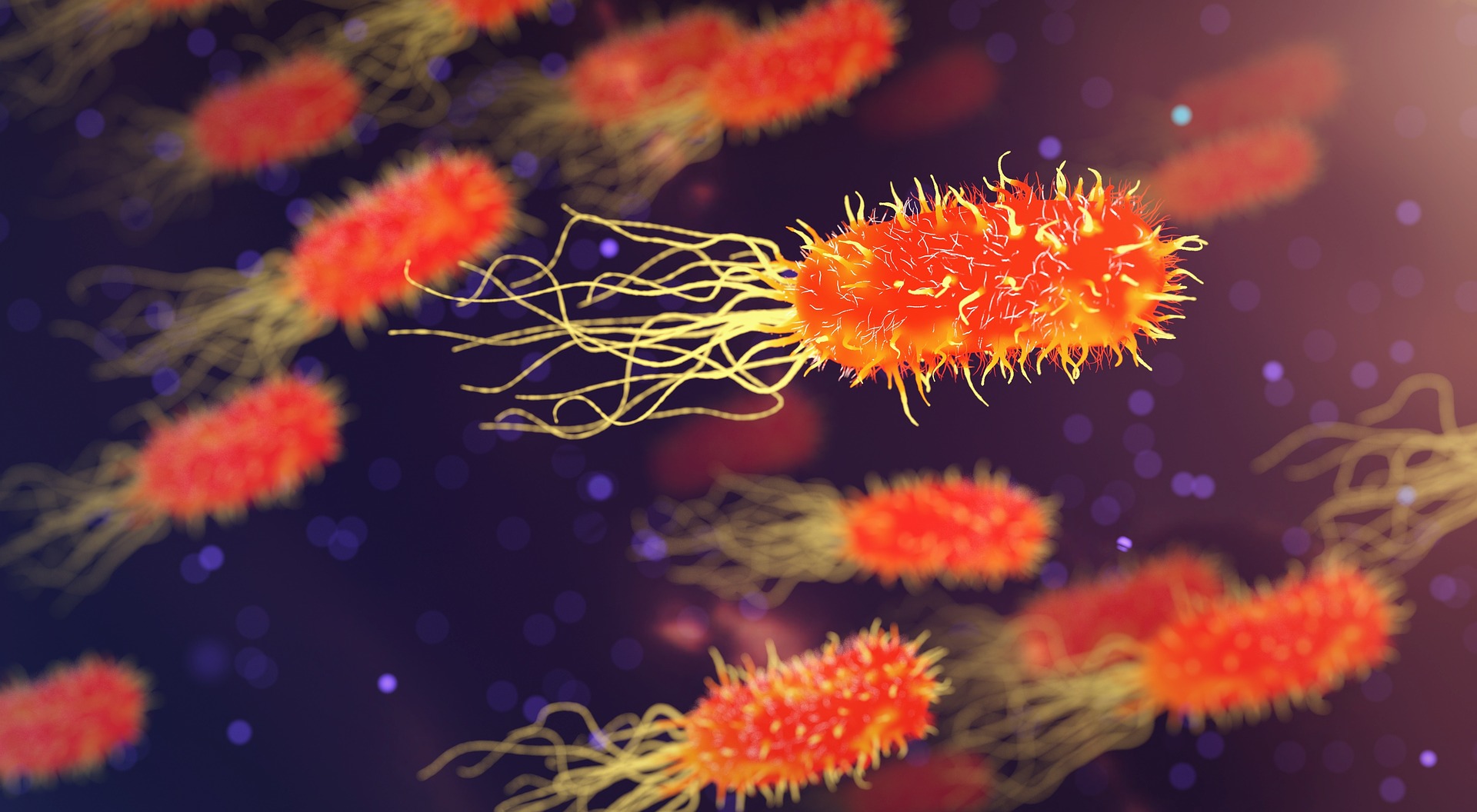
My work in this field has mainly made use of a relatively simple design of a microswimmer -- the bead-spring model -- in order to throw light on several fundamental properties of microswimming. Using this model we have suggested a possible explanation for why bacteria sometimes swim faster in fluids of higher viscosity, and described the effects of increased smoothness and deformability of a swimmer's surface on its velocity.
See further:
Setting the pace of microswimmers: when increasing viscosity speeds up self-propulsion, Jayant, L. Merchant, T. Krüger, J. Harting and A.-S. Smith, New Journal of Physics 19, 053024 (2017). DOI:10.1088/1367-2630/aa6e3a
Forces and shapes as determinants of micro-swimming: effect on synchronization and the utilization of drag, Jayant and A.-S. Smith, Soft Matter 11, 2364 (2015). DOI:10.1039/C4SM02611J
Effect of body deformability on microswimming, Jayant, L. Merchant, T. Krüger, J. Harting and A.-S. Smith, Soft Matter 13, 3984 (2017). DOI:10.1039/C7SM00181A
Colloidal motion in liquid crystals
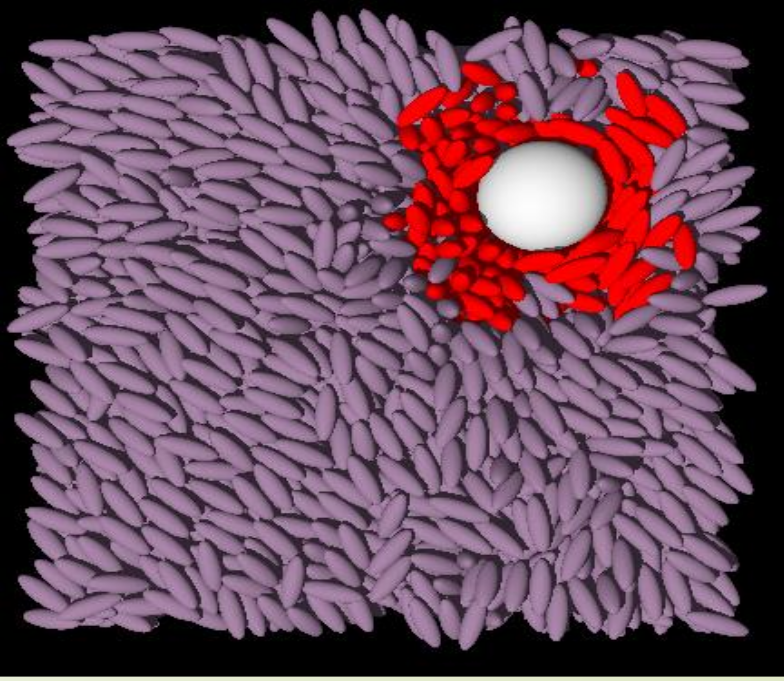
My work on LCs has focused on the properties of active and passive colloidal motion within them, in order to study both the evolution of LC phases and the properties of colloidal motion in heterogenous environments. In ongoing work, we are studying the genesis of defects in a nematic LC depending on the activity of an immersed colloidal particle, and the effect of different kinds of LC anchoring on the colloid's motion.